If you wish to contribute or participate in the discussions about articles you are invited to contact the Editor
Galileo Space Segment: Difference between revisions
No edit summary |
mNo edit summary |
||
(80 intermediate revisions by 9 users not shown) | |||
Line 1: | Line 1: | ||
{{Article Infobox2 | {{Article Infobox2 | ||
|Category=GALILEO | |Category=GALILEO | ||
| | |Editors=GMV | ||
|Level=Basic | |Level=Basic | ||
|YearOfPublication=2011 | |YearOfPublication=2011 | ||
|Logo=GMV | |Logo=GMV | ||
|Title={{PAGENAME}} | |||
}} | }} | ||
The Galileo | The Galileo constellation comprises of 30 satellites placed in MEO orbit, with 10 satellites placed in each of 3 orbital planes (at 56º nominal inclination <ref name="Galileo_Early_Services">[https://www.gsc-europa.eu/galileo/system Galileo System in European GNSS Service Centre website]</ref>) distributed evenly round the equator. The active constellation comprises of 24 satellites (Walker 24/3/1), including 6 spare satellites, which can be moved to replace any failed satellite within the same plane, thereby reducing the impact of failures upon quality of service. All satellites are identical in terms of design, performance capability and fuel load. Each satellite broadcasts navigation timing signals together with navigation data providing the clock and ephemeris correction data which are essential for navigation.<ref name="Galileo_Early_Services">[https://www.gsc-europa.eu/galileo/system Galileo System in European GNSS Service Centre website]</ref> | ||
The orbit altitude of 23 222 km (orbital radius: 29994 Km) results in an orbit of repeated trace with respect to the orientation of the Earth below them, that repeats itself after ten days during which each satellite has completed seventeen revolutions. | |||
==Constellation features== | ==Constellation features== | ||
[[File:Galileo Space Segment.jpg|left|thumb|400px|Galileo Space Segment]] | [[File:Galileo Space Segment.jpg|left|thumb|400px|Galileo Space Segment]] | ||
The altitude of the satellites has been chosen to avoid gravitational resonances so that, after initial orbit optimisation, station-keeping manoeuvres will not be needed during the lifetime of a satellite. The altitude chosen also ensures a high visibility of the satellites. | The altitude of the satellites has been chosen to avoid gravitational resonances so that, after initial orbit optimisation, station-keeping manoeuvres will not be needed during the lifetime of a satellite. The altitude chosen also ensures a high visibility of the satellites. | ||
Line 19: | Line 19: | ||
The position constraints for individual satellites are set by the need to maintain a uniform constellation, for which it is specified that each satellite should be within +/- 2° of its nominal position relative to the adjacent satellites in the same orbit plane and should be within 2° of the orbit plane. | The position constraints for individual satellites are set by the need to maintain a uniform constellation, for which it is specified that each satellite should be within +/- 2° of its nominal position relative to the adjacent satellites in the same orbit plane and should be within 2° of the orbit plane. | ||
The in-plane accuracy is equivalent to a relative tolerance of over 1000 km but requires very careful adjustment of the satellite velocity to ensure that the orbit period of all the satellites is kept precisely the same. The across-track tolerance allows the inclination and RAAN of each satellite to be biased at launch so that natural drifts remain within the tolerance without the need for orbit plane changes requiring major expense of fuel. | The in-plane accuracy is equivalent to a relative tolerance of over 1000 km but requires very careful adjustment of the satellite velocity to ensure that the orbit period of all the satellites is kept precisely the same. The across-track tolerance allows the inclination and Right Ascension of the Ascending Node (RAAN) of each satellite to be biased at launch so that natural drifts remain within the tolerance without the need for orbit plane changes requiring major expense of fuel. | ||
The spare satellite in each orbit plane ensures that in case of failure the constellation can be repaired quickly by moving the spare to replace the failed satellite. This could be done in a matter of days, rather than waiting for a new launch to be arranged which could take many months. The satellites are designed to be compatible with a range of launchers providing multiple and dual launch capabilities. | The spare satellite in each orbit plane ensures that in case of failure the constellation can be repaired quickly by moving the spare to replace the failed satellite. This could be done in a matter of days, rather than waiting for a new launch to be arranged which could take many months. The satellites are designed to be compatible with a range of launchers providing multiple and dual launch capabilities. | ||
There are good reasons for choosing such a structure for the Galileo constellation. With 30 satellites at such an altitude, there is a very high probability (more than 90%) that anyone anywhere in the world | There are good reasons for choosing such a structure for the Galileo constellation. With 30 satellites at such an altitude, there is a very high probability (more than 90%) that anyone anywhere in the world can always be in sight of at least four satellites and hence is able to determine their position from the ranging signals broadcast by the satellites. The inclination of the orbits was chosen to ensure good coverage of polar latitudes, which are poorly served by the US GPS system. | ||
From most locations, six to eight satellites | From most locations, six to eight satellites may always be visible, allowing positions to be determined very accurately – to within a few centimeters. Even in high rise cities, there is a good chance that a road user may have sufficient satellites overhead for taking a position, especially as the Galileo system is interoperable with the US system of 24 GPS satellites. | ||
This constellation provides good local geometries with a typical vertical dilution of precision (VDOP) of 2.3 and horizontal dilution of precision (HDOP) around 1.3. An additional benefit of the constellation geometry is the limited number of planes, which allows for faster deployment and reduced constellation maintenance costs due to the capability to launch multiple satellites with a single launcher. | |||
==Galileo satellites== | ==Galileo satellites== | ||
[[File:Galileo satellite system.jpg|right|thumb|300px|Galileo satellite]] | [[File:Galileo satellite system.jpg|right|thumb|300px|Galileo satellite]] | ||
The Galileo constellation is composed of a total of 30 Medium Earth Orbit (MEO) satellites, of which | The Galileo constellation is composed of a total of 30 Medium Earth Orbit (MEO) satellites, of which 6 are spares. Each satellite broadcasts precise time signals, ephemeris and other data. The Galileo satellite constellation has been optimised to the following nominal constellation specifications:<ref name="OS SDD"> [https://www.gsc-europa.eu/sites/default/files/sites/all/files/Galileo-OS-SDD_v1.1.pdf Applicable galileo Open Service (OS) Service Definition Document (SDD)]</ref> | ||
*circular orbits (satellite altitude of 23 222 km) | *circular orbits (satellite altitude of 23 222 km) | ||
*orbital inclination of 56° | *orbital inclination of 56° | ||
*three equally spaced orbital planes | *three equally spaced orbital planes | ||
* | *eight operational satellites, equally spaced in each plane | ||
* | *six spare satellites (also transmitting) | ||
The constellation is complemented by spare satellites that can be repositioned to any given slot depending on maintenance or service evolution needs. The location of the spare satellites in each plane is not yet frozen and will be decided at the time of deployment of the spare capability.<ref name="OS SDD"> [https://www.gsc-europa.eu/sites/default/files/sites/all/files/Galileo-OS-SDD_v1.1.pdf Applicable galileo Open Service (OS) Service Definition Document (SDD)]</ref> | |||
Each Galileo satellite is a 700 kg/1600 W class satellite, contains all the equipment needed to perform its assigned navigation and timing tasks over the course of its 12-year design life. Powered by solar arrays, with its internal components carefully shielded against the radiation prevailing in medium-Earth orbit. | |||
The image shows an artist's impression of a Galileo spacecraft in orbit with solar arrays deployed. The spacecraft rotates about its Earth-pointing axis so that the flat surface of the solar arrays always faces the Sun to collect maximum solar energy. The antennas, shown on the underside of the body in the picture, always point towards the Earth. The spacecraft body will measure 2.7 m x 1.1 m x 1.2 m and the deployed solar arrays span 13 m. | The image shows an artist's impression of a Galileo spacecraft in orbit with solar arrays deployed. The spacecraft rotates about its Earth-pointing axis so that the flat surface of the solar arrays always faces the Sun to collect maximum solar energy. The antennas, shown on the underside of the body in the picture, always point towards the Earth. The spacecraft body will measure 2.7 m x 1.1 m x 1.2 m and the deployed solar arrays span 13 m. | ||
===Galileo Satellite subsystems=== | |||
The satellite is composed of the following subsystems: | |||
*Payload Subsystem including the navigation payload and the SAR payload | |||
*Structure Subsystem | |||
*Thermal Control Subsystem (TCS) | |||
*Electrical Power Subsystem (EPS) with the following units: | |||
**Solar Arrays (SA) | |||
**Solar Array Drive Mechanisms (SADM) | |||
**Battery | |||
**Power Conditioning and Distribution Unit (PCDU) | |||
*Harness | |||
*Avionics Subsystem with | |||
**on-board computer (Integrated Control and Data Handling Unit, ICDU) | |||
**Attitude and Orbit Control System, AOCS (based on earth sensors, sun sensors, gyros, reaction wheels and magnetic torquers), | |||
**Software (SW) | |||
*Telemetry, Tracking and Command (TTC) Subsystem (with S-Band Transponder and two low-gain, omni-directional antennas) | |||
*Propulsion Subsystem (mono-propellant system with one tank and 8 thrusters) | |||
*Laser Retro-Reflector (LRR) | |||
*Platform Security Unit (PFSU) | |||
===Galileo Satellite components=== | ===Galileo Satellite components=== | ||
The '''L-band antenna''' transmits the navigation signals in the 1200-1600 MHz frequency range. | The '''L-band antenna''' transmits the navigation signals in the 1200-1600 MHz frequency range. | ||
The '''SAR (Search and Rescue) antenna''' picks up distress signals from beacons on Earth and transmits them to a ground station for forwarding to local rescue services. | The '''[[Galileo Search and Rescue Service|SAR (Search and Rescue) antenna]]''' picks up distress signals from beacons on Earth and transmits them to a ground station for forwarding to local rescue services. | ||
The '''C-band antenna''' receives signals containing mission data from Galileo Uplink Stations. This includes data to synchronise the on-board clocks with a ground-based reference clock and integrity data which contains information about how well each satellite is functioning. The integrity information is incorporated into the navigation signal for transmission to users. | The '''C-band antenna''' receives signals containing mission data from Galileo Uplink Stations. This includes data to synchronise the on-board clocks with a ground-based reference clock and integrity data which contains information about how well each satellite is functioning. The integrity information is incorporated into the navigation signal for transmission to users. | ||
Line 54: | Line 79: | ||
The '''laser retro-reflector''' allows the measurement of the satellite's altitude to within a few centimetres by reflecting a laser beam transmitted by a ground station. The laser retro-reflector is used only about once a year, as altitude measurements via S-band antenna ranging signals are otherwise accurate enough. | The '''laser retro-reflector''' allows the measurement of the satellite's altitude to within a few centimetres by reflecting a laser beam transmitted by a ground station. The laser retro-reflector is used only about once a year, as altitude measurements via S-band antenna ranging signals are otherwise accurate enough. | ||
The '''space radiators''' are heat exchangers that radiate waste heat, produced by the units inside the spacecraft, to deep space and thus help to keep the units within their operational temperature range. | The '''space radiators''' are heat exchangers that radiate waste heat, produced by the units inside the spacecraft, to deep space and thus help to keep the units within their operational temperature range. | ||
In the following image are identified the main external components: <ref name = "Satellite anatomy">[https://www.esa.int/Applications/Navigation/Galileo/Satellite_anatomy "Satellite anatomy”, ESA] </ref> | |||
[[File:ExternalPartsGalileoSatellite.png | none|thumb|400px| Source: "Satellite anatomy”, ESA<ref name = "Satellite anatomy">[https://www.esa.int/Applications/Navigation/Galileo/Satellite_anatomy "Satellite anatomy”, ESA] </ref>]] | |||
===Interior: payload=== | ===Interior: payload=== | ||
The Galileo satellites include two payloads, the Navigation payload and the Search and Rescue payload. | |||
Their main functions are: | |||
*Provision of on-board timing signals | |||
*Receipt & storage of up-linked navigation message data | |||
*Receipt & storage of up-linked integrity data | |||
*Assembly of navigation message in the agreed format | |||
*Error correction coding of navigation message | |||
*Generation of ranging codes | |||
*Encryption of ranging codes as required | |||
*Generation and modulation of L-Band carrier signals | |||
*Broadcast of navigation signals | |||
The timing signals are provided by high precision on-board clocks, implemented as two (cold) redundant pairs per satellite, each pair including two different technologies, both of them being operated simultaneously: | |||
A '''Passive Hydrogen Maser (PHM) clock''' is the master clock on board the spacecraft. It is an atomic clock which uses the ultra stable 1.4 GHz transition in a hydrogen atom to measure time to within 0.45 ns over 12 hours. | |||
A '''Rubidium Atomic Frequency Standard (RAFS) clock''' will be used should the maser clock fail. It is accurate to within 1.8 ns over 12 hours. | |||
[[File:Rubidium_clock.jpg|left|thumb|250px|Rubidium clock]] | |||
At any time, only one clock of each type is operating. Under normal conditions, the operating maser clock produces the reference frequency from which the navigation signal is generated. Should the maser clock fail, however, the operating rubidium clock will take over instantaneously and the two reserve clocks will start up. If the problem with the failed maser clock is unique to that clock, the second maser clock will take over from the rubidium clock after a few days when it is fully operational. The rubidium clock will then go on stand-by or reserve again. In this way, by having four clocks, the Galileo spacecraft is guaranteed to generate a navigation signal at all times. | |||
The '''clock monitoring and control unit''' provides the interface between the four clocks and the navigation signal generator unit (NSGU). It passes the signal from the active master clock to the NSGU and also ensures that the frequencies produced by the master clock and the active spare are in phase, so that the spare can take over instantly should the master clock fail. | |||
The '''navigation signal generator, frequency generator and up-conversion units''' are in charge of generating the navigation signals using input from the clock monitoring unit and the up-linked navigation and integrity data from the C-band antenna. The navigation signals are converted to L-band for broadcast to users. | |||
The '''navigation signal generator, frequency generator and up-conversion units (NSGU)''', which includes internal cold redundancy, receives the up-linked navigation data and uses them to generate the navigation signals in the appropriate format, performs the PRN encoding and the modulation of the 3 navigation signals (E5a + E5b, E6 and L1) and passes them to the Frequency Generation and Up-conversion Unit (FGUU) which performs the up- conversion into L-band of the 3 signals. | |||
The '''remote terminal unit''' is the interface between all the payload units and the on-board computer. | |||
===Interior: service module=== | |||
'''SADM''' is the drive mechanism that connects the solar arrays to the spacecraft and rotates them slowly so that the surface of the arrays can remain perpendicular to the Sun's rays at all times. | |||
The '''gyroscopes''' measure the rotation of the spacecraft. | |||
The '''reaction wheels''' control the rotation of the spacecraft. When they rotate, so does the spacecraft. It rotates twice per orbit to allow the solar arrays to remain parallel to the Sun's rays. | |||
The '''magneto bar''' modifies the speed of rotation of the reaction wheels by introducing a torque (turning force) in the opposite direction. | |||
The '''power conditioning and distribution unit''' regulates and controls power from the solar arrays and batteries and distributes it to all the spacecraft's subsystems and payload. | |||
The '''environmental monitoring unit''' on-board takes radiation measurements. | |||
The '''on-board computer''' controls all aspects of spacecraft and payload functioning. | |||
=== Satellites Key Features<ref>[https://www.ohb-system.de/files/images/mediathek/downloads/190603_OHB-System_Galileo_FOC-Satellites_2019-05.pdf Key Features of the Galileo Satellites], OHB</ref> === | |||
{| class="wikitable" | |||
|- | |||
! colspan="2" | Key Features | |||
|- | |||
|Body dimensions || 2.5 x 1.2 x 1.1 m | |||
|- | |||
|Span solar generator || 14.67 m | |||
|- | |||
|Solar generator power || 1.9 Kw | |||
|- | |||
|Navigation signals || 3 bands (E5, E6, E1) | |||
|- | |||
|SAR Transponder || UHF Receiver 406 MHz | |||
L-Band transceiver 1,544 MHz | |||
|- | |||
|Lifetime || > 12 years on-orbit | |||
> 5 years ground storage | |||
|- | |||
|Reliability || > 0.88 /12 years | |||
|- | |||
|Launch mass || 732.8 Kg | |||
|} | |||
==Galileo Metadata == | |||
The Galileo Metadata can be found in the GNSS Service Centre Web Page. See the following link: | |||
https://www.gsc-europa.eu/support-to-developers/galileo-satellite-metadata | |||
==Satellites Names and Launching == | |||
Up to date information on the Galileo program, namely launching and phases can be found in: <ref>[https://www.gsc-europa.eu/system-service-status/constellation-information Current Galileo constellation status] </ref>. | |||
European Commission created a children's drawing competition to name each launched satellite. The competition was open to children from all member states and the name of the winner from each state was given to one of the Galileo satellites. | |||
The full list of the winners competition can be found [http://ec.europa.eu/enterprise/policies/satnav/galileo/drawing-competition/index_en.htm here], as well the drawings that gave them the victory. | |||
More information on the Galileo IOV satellites is provided [https://ec.europa.eu/growth/sectors/space/galileo/drawing-competition_en| here]. | |||
==References== | ==References== | ||
<references/> | <references/> | ||
[[Category:GALILEO]] | [[Category:GALILEO]] | ||
[[Category:GALILEO Architecture]] | |||
[[Category:GALILEO Space Segment]] |
Latest revision as of 12:26, 15 August 2024
GALILEO | |
---|---|
Title | Galileo Space Segment |
Edited by | GMV |
Level | Basic |
Year of Publication | 2011 |
The Galileo constellation comprises of 30 satellites placed in MEO orbit, with 10 satellites placed in each of 3 orbital planes (at 56º nominal inclination [1]) distributed evenly round the equator. The active constellation comprises of 24 satellites (Walker 24/3/1), including 6 spare satellites, which can be moved to replace any failed satellite within the same plane, thereby reducing the impact of failures upon quality of service. All satellites are identical in terms of design, performance capability and fuel load. Each satellite broadcasts navigation timing signals together with navigation data providing the clock and ephemeris correction data which are essential for navigation.[1]
The orbit altitude of 23 222 km (orbital radius: 29994 Km) results in an orbit of repeated trace with respect to the orientation of the Earth below them, that repeats itself after ten days during which each satellite has completed seventeen revolutions.
Constellation features
The altitude of the satellites has been chosen to avoid gravitational resonances so that, after initial orbit optimisation, station-keeping manoeuvres will not be needed during the lifetime of a satellite. The altitude chosen also ensures a high visibility of the satellites.
The position constraints for individual satellites are set by the need to maintain a uniform constellation, for which it is specified that each satellite should be within +/- 2° of its nominal position relative to the adjacent satellites in the same orbit plane and should be within 2° of the orbit plane.
The in-plane accuracy is equivalent to a relative tolerance of over 1000 km but requires very careful adjustment of the satellite velocity to ensure that the orbit period of all the satellites is kept precisely the same. The across-track tolerance allows the inclination and Right Ascension of the Ascending Node (RAAN) of each satellite to be biased at launch so that natural drifts remain within the tolerance without the need for orbit plane changes requiring major expense of fuel.
The spare satellite in each orbit plane ensures that in case of failure the constellation can be repaired quickly by moving the spare to replace the failed satellite. This could be done in a matter of days, rather than waiting for a new launch to be arranged which could take many months. The satellites are designed to be compatible with a range of launchers providing multiple and dual launch capabilities.
There are good reasons for choosing such a structure for the Galileo constellation. With 30 satellites at such an altitude, there is a very high probability (more than 90%) that anyone anywhere in the world can always be in sight of at least four satellites and hence is able to determine their position from the ranging signals broadcast by the satellites. The inclination of the orbits was chosen to ensure good coverage of polar latitudes, which are poorly served by the US GPS system.
From most locations, six to eight satellites may always be visible, allowing positions to be determined very accurately – to within a few centimeters. Even in high rise cities, there is a good chance that a road user may have sufficient satellites overhead for taking a position, especially as the Galileo system is interoperable with the US system of 24 GPS satellites.
This constellation provides good local geometries with a typical vertical dilution of precision (VDOP) of 2.3 and horizontal dilution of precision (HDOP) around 1.3. An additional benefit of the constellation geometry is the limited number of planes, which allows for faster deployment and reduced constellation maintenance costs due to the capability to launch multiple satellites with a single launcher.
Galileo satellites
The Galileo constellation is composed of a total of 30 Medium Earth Orbit (MEO) satellites, of which 6 are spares. Each satellite broadcasts precise time signals, ephemeris and other data. The Galileo satellite constellation has been optimised to the following nominal constellation specifications:[2]
- circular orbits (satellite altitude of 23 222 km)
- orbital inclination of 56°
- three equally spaced orbital planes
- eight operational satellites, equally spaced in each plane
- six spare satellites (also transmitting)
The constellation is complemented by spare satellites that can be repositioned to any given slot depending on maintenance or service evolution needs. The location of the spare satellites in each plane is not yet frozen and will be decided at the time of deployment of the spare capability.[2]
Each Galileo satellite is a 700 kg/1600 W class satellite, contains all the equipment needed to perform its assigned navigation and timing tasks over the course of its 12-year design life. Powered by solar arrays, with its internal components carefully shielded against the radiation prevailing in medium-Earth orbit.
The image shows an artist's impression of a Galileo spacecraft in orbit with solar arrays deployed. The spacecraft rotates about its Earth-pointing axis so that the flat surface of the solar arrays always faces the Sun to collect maximum solar energy. The antennas, shown on the underside of the body in the picture, always point towards the Earth. The spacecraft body will measure 2.7 m x 1.1 m x 1.2 m and the deployed solar arrays span 13 m.
Galileo Satellite subsystems
The satellite is composed of the following subsystems:
- Payload Subsystem including the navigation payload and the SAR payload
- Structure Subsystem
- Thermal Control Subsystem (TCS)
- Electrical Power Subsystem (EPS) with the following units:
- Solar Arrays (SA)
- Solar Array Drive Mechanisms (SADM)
- Battery
- Power Conditioning and Distribution Unit (PCDU)
- Harness
- Avionics Subsystem with
- on-board computer (Integrated Control and Data Handling Unit, ICDU)
- Attitude and Orbit Control System, AOCS (based on earth sensors, sun sensors, gyros, reaction wheels and magnetic torquers),
- Software (SW)
- Telemetry, Tracking and Command (TTC) Subsystem (with S-Band Transponder and two low-gain, omni-directional antennas)
- Propulsion Subsystem (mono-propellant system with one tank and 8 thrusters)
- Laser Retro-Reflector (LRR)
- Platform Security Unit (PFSU)
Galileo Satellite components
The L-band antenna transmits the navigation signals in the 1200-1600 MHz frequency range.
The SAR (Search and Rescue) antenna picks up distress signals from beacons on Earth and transmits them to a ground station for forwarding to local rescue services.
The C-band antenna receives signals containing mission data from Galileo Uplink Stations. This includes data to synchronise the on-board clocks with a ground-based reference clock and integrity data which contains information about how well each satellite is functioning. The integrity information is incorporated into the navigation signal for transmission to users.
Two S-band antennas are part of the telemetry, tracking and command subsystem. They transmit housekeeping data about the payload and spacecraft to ground control and, in turn, receive commands to control the spacecraft and operate the payload. The S-band antennas also receive, process and transmit ranging signals that measure the satellite's altitude to within a few metres.
The infrared Earth sensors and the Sun sensors both help to keep the spacecraft pointing at the Earth. The infrared Earth sensors do this by detecting the contrast between the cold of deep space and the heat of the Earth's atmosphere. The Sun sensors are visible light detectors which measure angles between their mounting base and incident sunlight.
The laser retro-reflector allows the measurement of the satellite's altitude to within a few centimetres by reflecting a laser beam transmitted by a ground station. The laser retro-reflector is used only about once a year, as altitude measurements via S-band antenna ranging signals are otherwise accurate enough.
The space radiators are heat exchangers that radiate waste heat, produced by the units inside the spacecraft, to deep space and thus help to keep the units within their operational temperature range.
In the following image are identified the main external components: [3]
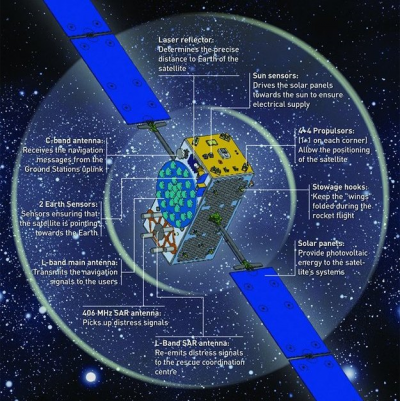
Interior: payload
The Galileo satellites include two payloads, the Navigation payload and the Search and Rescue payload. Their main functions are:
- Provision of on-board timing signals
- Receipt & storage of up-linked navigation message data
- Receipt & storage of up-linked integrity data
- Assembly of navigation message in the agreed format
- Error correction coding of navigation message
- Generation of ranging codes
- Encryption of ranging codes as required
- Generation and modulation of L-Band carrier signals
- Broadcast of navigation signals
The timing signals are provided by high precision on-board clocks, implemented as two (cold) redundant pairs per satellite, each pair including two different technologies, both of them being operated simultaneously:
A Passive Hydrogen Maser (PHM) clock is the master clock on board the spacecraft. It is an atomic clock which uses the ultra stable 1.4 GHz transition in a hydrogen atom to measure time to within 0.45 ns over 12 hours.
A Rubidium Atomic Frequency Standard (RAFS) clock will be used should the maser clock fail. It is accurate to within 1.8 ns over 12 hours.
At any time, only one clock of each type is operating. Under normal conditions, the operating maser clock produces the reference frequency from which the navigation signal is generated. Should the maser clock fail, however, the operating rubidium clock will take over instantaneously and the two reserve clocks will start up. If the problem with the failed maser clock is unique to that clock, the second maser clock will take over from the rubidium clock after a few days when it is fully operational. The rubidium clock will then go on stand-by or reserve again. In this way, by having four clocks, the Galileo spacecraft is guaranteed to generate a navigation signal at all times.
The clock monitoring and control unit provides the interface between the four clocks and the navigation signal generator unit (NSGU). It passes the signal from the active master clock to the NSGU and also ensures that the frequencies produced by the master clock and the active spare are in phase, so that the spare can take over instantly should the master clock fail.
The navigation signal generator, frequency generator and up-conversion units are in charge of generating the navigation signals using input from the clock monitoring unit and the up-linked navigation and integrity data from the C-band antenna. The navigation signals are converted to L-band for broadcast to users.
The navigation signal generator, frequency generator and up-conversion units (NSGU), which includes internal cold redundancy, receives the up-linked navigation data and uses them to generate the navigation signals in the appropriate format, performs the PRN encoding and the modulation of the 3 navigation signals (E5a + E5b, E6 and L1) and passes them to the Frequency Generation and Up-conversion Unit (FGUU) which performs the up- conversion into L-band of the 3 signals.
The remote terminal unit is the interface between all the payload units and the on-board computer.
Interior: service module
SADM is the drive mechanism that connects the solar arrays to the spacecraft and rotates them slowly so that the surface of the arrays can remain perpendicular to the Sun's rays at all times.
The gyroscopes measure the rotation of the spacecraft.
The reaction wheels control the rotation of the spacecraft. When they rotate, so does the spacecraft. It rotates twice per orbit to allow the solar arrays to remain parallel to the Sun's rays.
The magneto bar modifies the speed of rotation of the reaction wheels by introducing a torque (turning force) in the opposite direction.
The power conditioning and distribution unit regulates and controls power from the solar arrays and batteries and distributes it to all the spacecraft's subsystems and payload.
The environmental monitoring unit on-board takes radiation measurements.
The on-board computer controls all aspects of spacecraft and payload functioning.
Satellites Key Features[4]
Key Features | |
---|---|
Body dimensions | 2.5 x 1.2 x 1.1 m |
Span solar generator | 14.67 m |
Solar generator power | 1.9 Kw |
Navigation signals | 3 bands (E5, E6, E1) |
SAR Transponder | UHF Receiver 406 MHz
L-Band transceiver 1,544 MHz |
Lifetime | > 12 years on-orbit
> 5 years ground storage |
Reliability | > 0.88 /12 years |
Launch mass | 732.8 Kg |
Galileo Metadata
The Galileo Metadata can be found in the GNSS Service Centre Web Page. See the following link:
https://www.gsc-europa.eu/support-to-developers/galileo-satellite-metadata
Satellites Names and Launching
Up to date information on the Galileo program, namely launching and phases can be found in: [5].
European Commission created a children's drawing competition to name each launched satellite. The competition was open to children from all member states and the name of the winner from each state was given to one of the Galileo satellites.
The full list of the winners competition can be found here, as well the drawings that gave them the victory.
More information on the Galileo IOV satellites is provided here.